Carbon Control in MIM
Introduction
As carbon control is the fundamental factor for final component quality, the challenge of carbon element has strong influence in MIM technology. The carbon controlling is involved in both sintering and debinding process of MIM cycle. The key parameters are sintering atmospheres and binder systems, MIM powders.
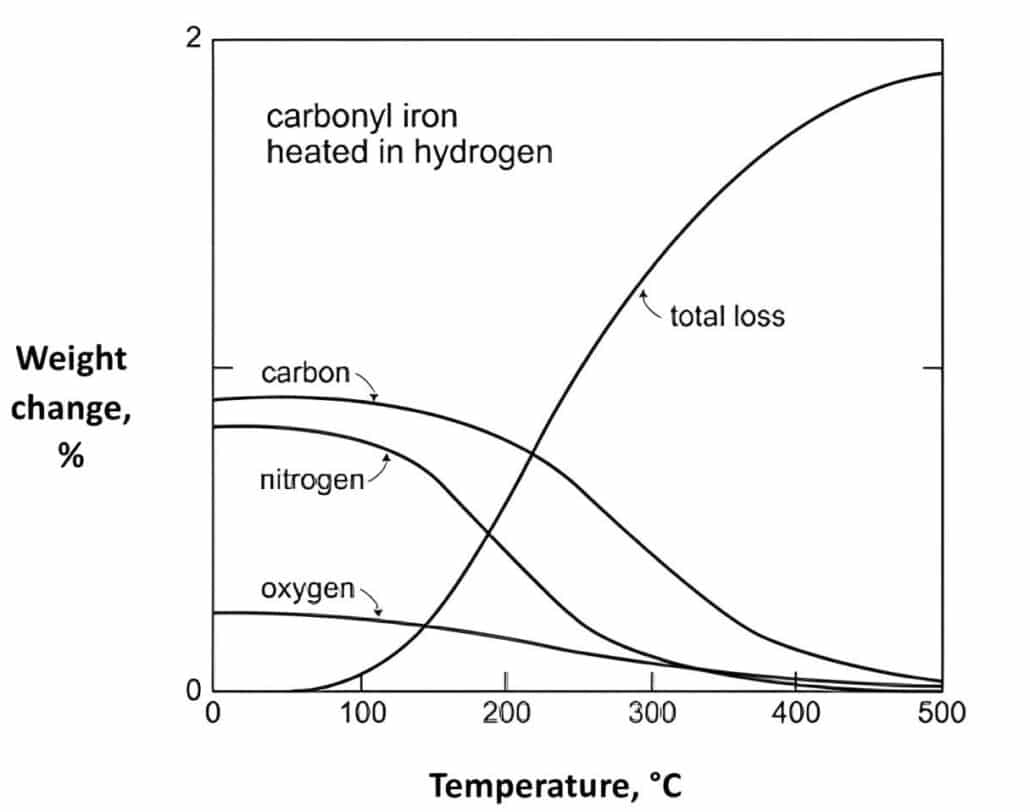
The final carbon levels depend on the final chemical requirement of final products, such as: less than 300 ppm for austenitic stainless steels, more than 5-6% for cemented carbides, various steels and tool steels with target carbon levels from 0.4% to 2%.
Carbonyl Iron Powder
In MIM technology, the focus of carbon controlling is shifting from ferrous alloys to more reactive material systems, like titanium, niobium, molybdenum. As rapid commercial development of MIM technology, Most carbon controlling issues in ferrous alloys are resolved, such as cemented carbides in tungsten alloys, aluminides, tool steels, stainless steels, and low alloy steels.
The following graph demonstrates the pearlite micro-structure after 1300℃ sintering, the final carbon level is at the eutectoid composition of 0.77 wt%.
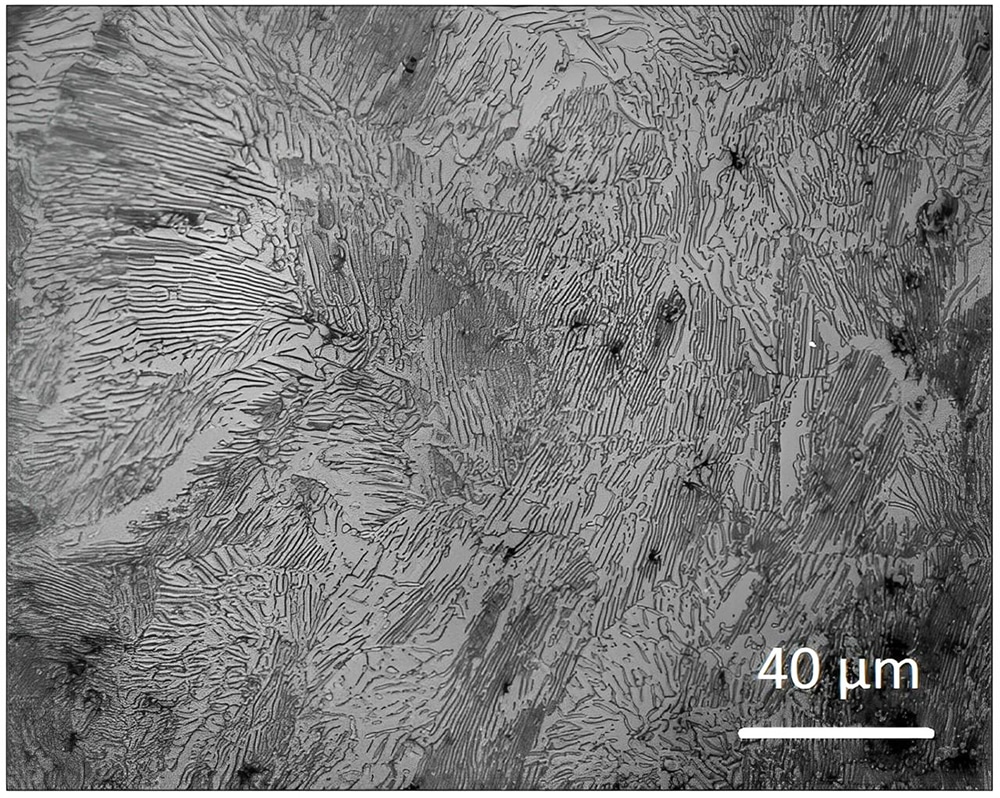
The solvent & catalytic debinding provide the manipulation of final carbon levels. The carbon composition is adjusted by decarburization during sintering, mostly are compensate oxygen in the powder. Experimental data determine the weight percent of oxygen in powder will the same weight percent carbon loss.
Atmosphere Basics
The final carbon level are determined by various elements in MIM, including MIM powder, sinering furnace operation, binder composition, sintering atmosphere. The sintering atmosphere is critical in several performance roles, the most important is protecting the compact from oxidation at high temperatures. Further, the atmosphere also can remove the burnout products.
Therefore, manipulations of carbon in MIM sintering are possible via atmosphere control. The common reactions of atmosphere during sintering include:
- Oxidization (carbon dioxide, oxygen)
- Reduction (hydrogen, carbon monoxide)
- Nitridation (nitrogen, ammonia)
- Carburization (methane)
- Decarburization (carbon dioxide, steam)
In Ferrous MIM sintering, the typical atmosphere choices are vacuum, hydrogen, nitrogen-hydrogen. Other combination is Argon-hydrogen.
Hydrogen
Hydrogen is superb for most ferrous alloys in sintering. It is soluble slightly in most ferrous alloys. For stainless steel with 12-20 wt.% chromium, hydrogen can reduce the normal chromium oxide on the particle surface.
Hydrogen sintering can improve the corrosion resistance, as it suppresses chromium evaporation. Chromium has higher vapor pressure than nickel and iron, it evaporates in vacuum sintering. Hydrogen reduces the surface oxides, create strong metallurgical bonds, then suppress evaporation kinetics and improve chromium retention. The chromium will reform the surface oxide once exposure to air, this transparent and light layer is tenacious to prevent corrosion. Therefore, pure hydrogen is the ideal sintering atmosphere for stainless steels.
Nitrogen
Nitrogen is neutral and beneficial for most ferrous alloys. It is an interstitial as carbon to increase strength. MIM steels with carbide additions and nitrogen atmosphere sintering can reach 3,000 MPa tensile strength with 3% elongation. The normal nitrogen atmosphere is 80 to 97% nitrogen with controlled levels of methane or hydrogen.
Vacuum
Vacuum sintering is not devoid of atmosphere, it implies a reduced pressure. The vacuum pressure in sintering chamber is only one ten-thousandth of atmospheric pressure. Under controlled conditions, vacuum sintering is a clean, reproducible and nonreactive environment, neither carburising nor decarburising. Most MIM steels are easily sintered in vacuum.
Binder System
In heating the MIM components to sintering temperature, it is critical to extract the last backbone polymer without residual carbon. Once the backbone polymer is heating to high temperature without evaporation, the decomposition of binder will result in higher carbon level.
Most polymers will decompose into volatile molecules in heating process, such as methane, ethane, butane, propane, carbon monoxide, carbon dioxide and water. The decomposition start in the early heating cycle, peak near 450 ℃, then finish by 550 ℃.
The best practice to remove the backbone polymer is slow heating in early portion of sintering cycle. Then atmosphere turnover will remove the burnout products by adjusted gas flow. The best polymer extraction is in atmosphere is hydrogen, or nitrogen&hydrogen.
Our realistic solutions rely on the closed-loop control systems, which can read the furnace situation, adjust the composition and atmosphere flow, and improve burnout.
Critical Metallurgical Reactions
The critical metallurgical reactions in MIM process are oxidation-reduction and carburisation-decarburisation. The additional heat treatments can achieve the required hardness, wear resistance, strength.
Oxidation-Reduction
Thermo-chemical reaction is the fundamental to carbon control in MIM steels, the reaction involve Fe equilibrium between oxygen and the oxide.
Fe (s) + ½ O2 (g) ↔ FeO (s)
Iron (Fe) and iron oxide (FeO) are solid, and oxygen is gas. This is a reversible reaction, the high concentration of oxygen leads to iron oxide, while high temperature of iron oxide also results in pure iron and oxygen.
From the following graph, it exhibits the oxygen partial pressure effect on oxidation-reduction. In the typical sintering temperature, the reduction requires atmosphere with less than 10-13 parts of oxygen, which is achievable in most systems.
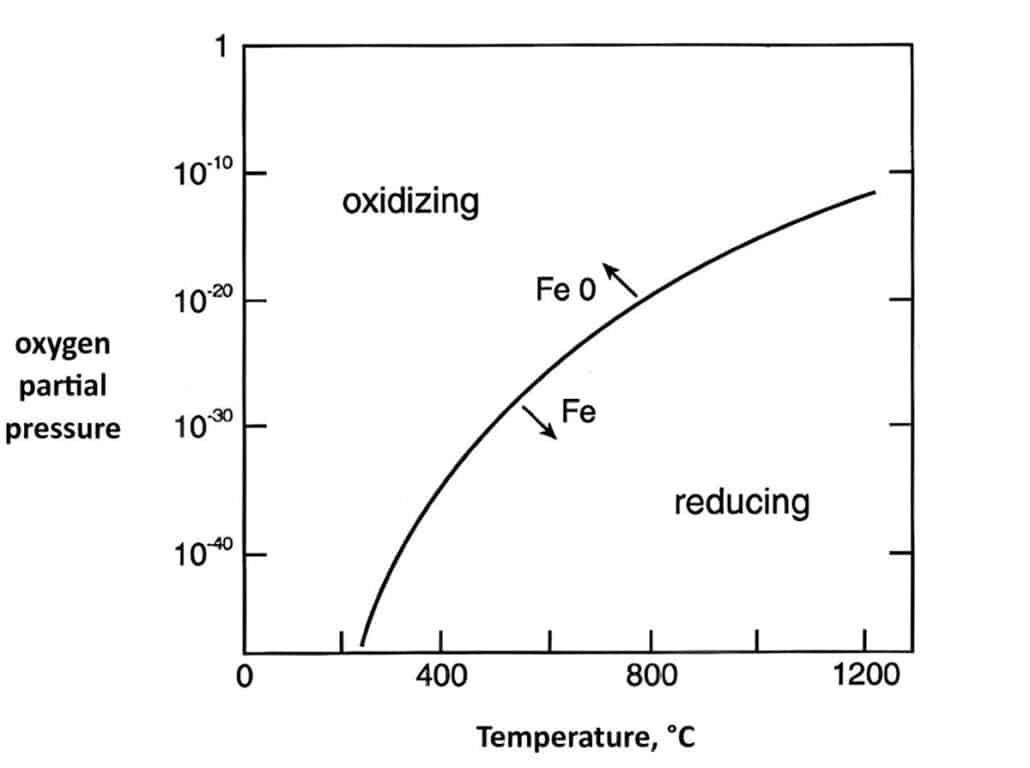
Higher sintering temperatures encourage reduction, especially with hydrogen. The reduction of solid Fe2O3:
Fe2O3 (s) + 3 H2 (g) ↔ 2 Fe (s) + 3 H2O (g)
The partial pressure ratio of hydrogen to water will determine the reaction progression. At 1200℃ sintering temperature, atmosphere with hydrogen to water pressure ratio over unity is favourable for reduction.
There are two effective reducing species in sintering ferrous alloys: carbon monoxide (CO) and hydrogen (H2).
FeO (s) + CO (g) ↔ Fe (s) + CO2 (g)
The partial pressure ratio of monoxide CO and carbon dioxide CO2 is critical in reaction.
Carburisation-Decarburisation
Carbon is a potent and necessary strengthening element in steels, the carburisation and decarburisation determine the precise control of final carbon content. Duo to the reactions between carbon, oxygen and hydrogen. Specific atmosphere is possible to add or subtract carbon from MIM parts. The common reaction are:
3 Fe (s) + 2 CO (g) ↔ Fe3C (s) + CO2 (g)
3 Fe (s) + CH4 (g) ↔ Fe3C (s) + 2 H2 (g)
The forming cementite Fe3C is in solution at low concentrations. The retained carbon and carbon dioxide content have relation for two temperatures as the follow graph.
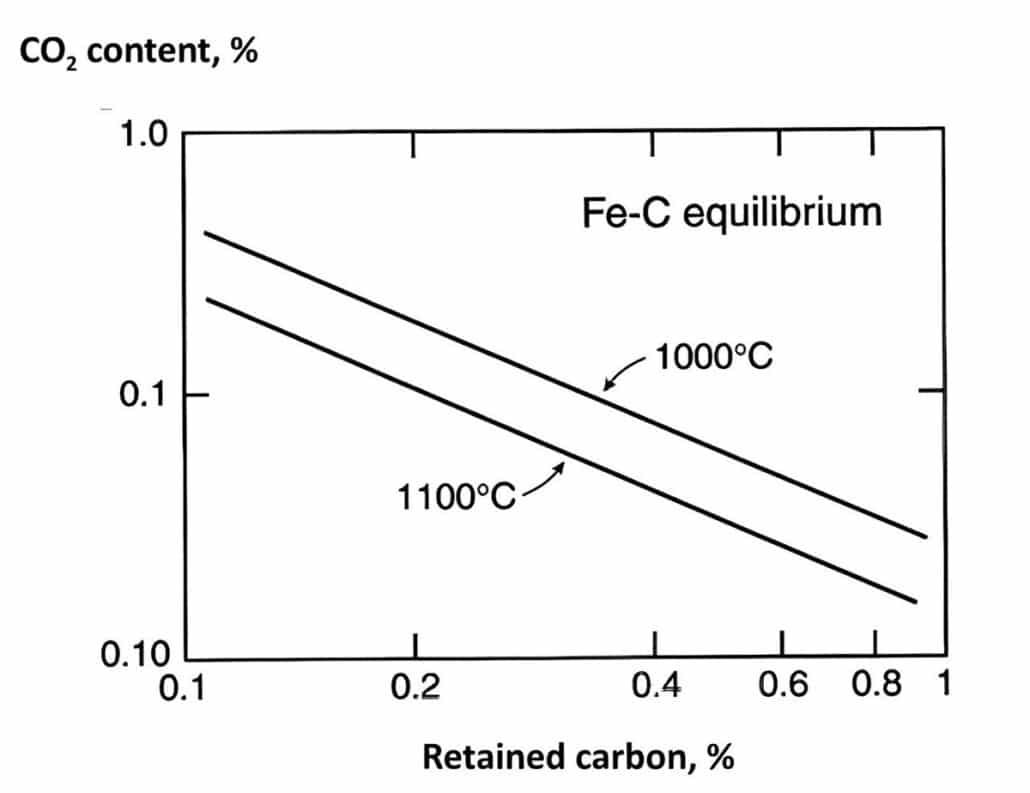
Higher retained carbon levels demonstrate the less carbon dioxide in atmosphere, the high carbon dioxide content also can drive decarburisation via carbon monoxide generation alternatively.
Vacuum sintering with graphite heaters result in reactions between residual oxygen and graphite to generate CO. Carbon monoxide has the most evident reduction at temperature over 800℃. While the carbon-based reduction of chromium oxide will occur until 1250℃. Graphite heating elements provide the carburizing environment for MIM sintering of tool steel.
Regardless the heating elements, carbon element can be introduced by the decomposed polymers or intentional graphite addition. The powder source of carbon include graphite and cementite (Fe3C), but contraindicate more thermodynamically stable carbides like chromium carbide.
Water Vapor (Steam)
The reaction between the carbide and water water vapor in sintering is
Fe3C (s) + H2O (g) ↔ 3 Fe (s) + CO (g) + H2 (g)
This indicates an increase in water pressure is one way to decarburize steel. Atmosphere with low water vapor level (dry or low dew points) can avoid carbon removal in sintering. But this slow decarburization is possible to generate methane, which is often ignored. Therefore, sintering in high purity hydrogen is the most desirable option of carbon retention.
Controlling Reactions
As there are five main gas species in MIM sintering atmosphere: CO, CO2, H2, H2O and CH4. We need to consider several reactions in carbon controlling of MIM steels. The two key reactions are:
CO2 (g) + H2 (g) ↔ CO (g) + H2O (g)
CH4 (g) + H2O (g) ↔ CO (g) + 3 H2 (g)
The full control of carbon reactions are adjusted by these species, quantitative measure of carbon is determined by the ratio of these gases.
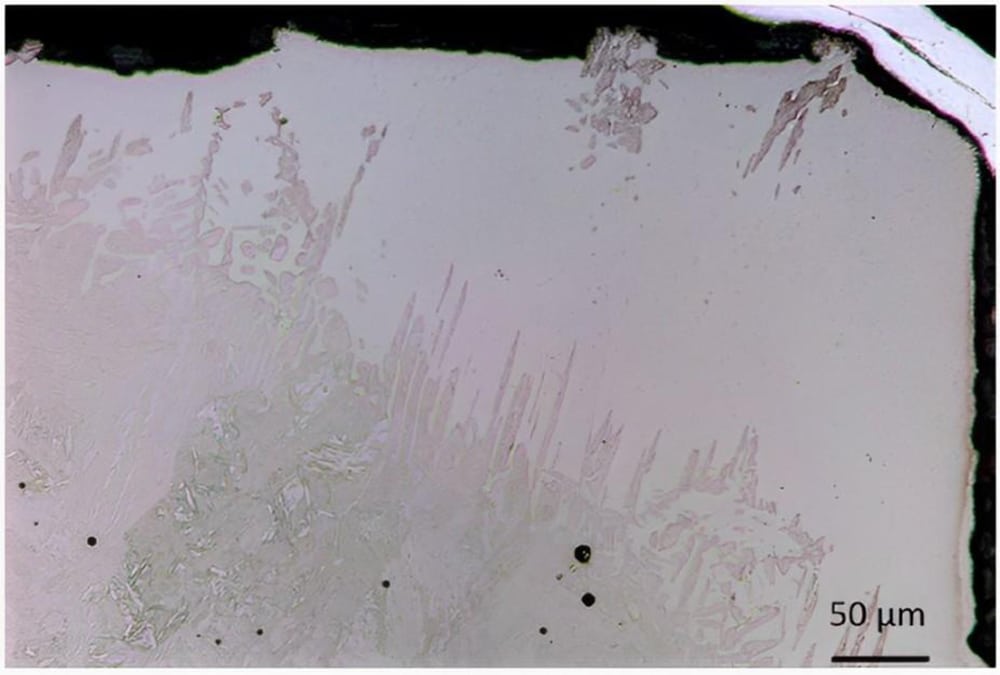
Conclusion
There are several factor impact the final carbon level in MIM parts. Power composition of the oxygen and carbon content dominate final MIM carbon levels. Additives of iron carbide or graphite powders can offset carbon loss from oxygen in the powder. The sintering atmosphere is another dominant factor. According to the oxidation-reduction and carburisation-decarburisation maps, we can adjust the atmosphere composition to achieve final carbon change.
In ZCMIM, we apply sensors and computer controls to adjust the process atmosphere, and achieve target carbon levels. For low final carbon levels, case caburization is an easier way to satisfy. Constantly monitor and control atmosphere quality is appropriate for intermediate carbon levels. Finally, for high carbon levels, the carbon should tie up into the stable carbides(SiC, WC, TiC, Cr2C3).
Ultimately, ZCMIM can provide precise carbon control with analytical sensors and atmosphere control algorithms. This enables our high volume applications for automotive, hand tool, medical, and industrial components. Contact us for your next MIM project.